Geometric and mechanical properties evaluation of scaffolds for bone tissue applications designing by a reaction-diffusion models and manufactured with a material jetting system
dc.contributor.author | Velasco, Marco A. | spa |
dc.contributor.author | Lancheros, Yadira | spa |
dc.contributor.author | Garzón-Alvarado, Diego A. | spa |
dc.coverage.campus | CRAI-USTA Bogotá | spa |
dc.date.accessioned | 2019-11-13T18:26:36Z | spa |
dc.date.available | 2019-11-13T18:26:36Z | spa |
dc.date.issued | 2016-10 | spa |
dc.description.abstract | Scaffolds are essential in bone tissue engineering, as they provide support to cells and growth factors necessary to regenerate tissue. In addition, they meet the mechanical function of the bone while it regenerates. Currently, the multiple methods for designing and manufacturing scaffolds are based on regular structures from a unit cell that repeats in a given domain. However, these methods do not resemble the actual structure of the trabecular bone which may work against osseous tissue regeneration. To explore the design of porous structures with similar mechanical properties to native bone, a geometric generation scheme from a reaction-diffusion model and its manufacturing via a material jetting system is proposed. This article presents the methodology used, the geometric characteristics and the modulus of elasticity of the scaffolds designed and manufactured. The method proposed shows its potential to generate structures that allow to control the basic scaffold properties for bone tissue engineering such as the width of the channels and porosity. The mechanical properties of our scaffolds are similar to trabecular tissue present in vertebrae and tibia bones. Tests on the manufactured scaffolds show that it is necessary to consider the orientation of the object relative to the printing system because the channel geometry, mechanical properties and roughness are heavily influenced by the position of the surface analyzed with respect to the printing axis. A possible line for future work may be the establishment of a set of guidelines to consider the effects of manufacturing processes in designing stages. | spa |
dc.description.domain | http://unidadinvestigacion.usta.edu.co | spa |
dc.format.mimetype | application/pdf | spa |
dc.identifier.doi | https://doi.org/10.1016/j.jcde.2016.06.006 | spa |
dc.identifier.uri | http://hdl.handle.net/11634/19743 | |
dc.relation.references | S. Weiner and H. D. Wagner, “The material bone: Structure-Mechanical Function Relations,” Annual Review of Materials Science, vol. 28, no. 1, pp. 271–298, Aug. 1998. | spa |
dc.relation.references | S. J. Morrison and D. T. Scadden, “The bone marrow niche for haematopoietic stem cells,” Nature, vol. 505, no. 7483, pp. 327–334, Jan. 2014. | spa |
dc.relation.references | J. F. Raposo, L. G. Sobrinho, and H. G. Ferreira, “A minimal mathematical model of calcium homeostasis,” The Journal of clinical endocrinology and metabolism, vol. 87, no. 9, pp. 4330–4340, Sep. 2002. | spa |
dc.relation.references | A. R. Amini, C. T. Laurencin, and S. P. Nukavarapu, “Bone tissue engineering: recent advances and challenges.,” Critical reviews in biomedical engineering, vol. 40, no. 5, pp. 363–408, Jan. 2012. | spa |
dc.relation.references | M. A. Velasco, C. A. Narváez-tovar, and D. A. Garzón-alvarado, “Design , Materials , and Mechanobiology of Biodegradable Scaffolds for Bone Tissue Engineering,” BioMed Research International, vol. 2015, no. Article ID 729076, p. 21, 2015. | spa |
dc.relation.references | Y. Ikada, “Challenges in tissue engineering,” Interface, vol. 3, no. 10, pp. 589–601, 2006. | spa |
dc.relation.references | K. J. Burg, S. Porter, and J. F. Kellam, “Biomaterial developments for bone tissue engineering.,” Biomaterials, vol. 21, no. 23, pp. 2347–59, Dec. 2000. | spa |
dc.relation.references | S. M. Giannitelli, D. Accoto, M. Trombetta, and A. Rainer, “Current trends in the design of scaffolds for computer-aided tissue engineering,” Acta biomaterialia, vol. 10, no. 2, pp. 580–594, Feb. 2014. | spa |
dc.relation.references | C. K. Chua, K. F. Leong, C. M. Cheah, and S. W. Chua, “Development of a Tissue Engineering Scaffold Structure Library for Rapid Prototyping . Part 2": Parametric Library and Assembly Program,” Advanced manufacturing technology, vol. 21, pp. 302–312, 2003. | spa |
dc.relation.references | N. Yang and K. Zhou, “Effective method for multi-scale gradient porous scaffold design and fabrication,” Materials science & engineering. C, Materials for biological applications, vol. 43, pp. 502–505, Oct. 2014. | spa |
dc.relation.references | A. Sutradhar and G. Paulino, “Topological optimization for designing patient-specific large craniofacial segmental bone replacements,” Proceedings of the National Academy of Sciences, vol. 107, no. 30, pp. 13222–13227, 2010. | spa |
dc.relation.references | P. Gonçalves Coelho, P. Rui Fernandes, and H. Carriço Rodrigues, “Multiscale modeling of bone tissue with surface and permeability control,” Journal of biomechanics, vol. 44, no. 2, pp. 321–329, Jan. 2011. | spa |
dc.relation.references | P. G. Coelho, S. J. Hollister, C. L. Flanagan, and P. R. Fernandes, “Bioresorbable scaffolds for bone tissue engineering: optimal design, fabrication, mechanical testing and scale-size effects analysis,” Medical engineering & physics, vol. 37, no. 3, pp. 287–296, Mar. 2015. | spa |
dc.relation.references | M. R. Dias, J. M. Guedes, C. L. Flanagan, S. J. Hollister, and P. R. Fernandes, “Optimization of scaffold design for bone tissue engineering: A computational and experimental study,” Medical engineering & physics, vol. 36, no. 4, pp. 448–457, Apr. 2014. | spa |
dc.relation.references | J. Woo Jung, H.-G. Yi, T.-Y. Kang, W.-J. Yong, S. Jin, W.-S. Yun, and D.-W. Cho, “Evaluation of the effective diffusivity of a freeform fabricated scaffold using computational simulation,” Journal of biomechanical engineering, vol. 135, no. 8, p. 7, Aug. 2013. | spa |
dc.relation.references | C. Provin, K. Takano, Y. Sakai, T. Fujii, and R. Shirakashi, “A method for the design of 3D scaffolds for high-density cell attachment and determination of optimum perfusion culture conditions,” Journal of Biomechanics, vol. 41, no. 7, pp. 1436–1449, 2008. | spa |
dc.relation.references | B. Starly and W. Sun, “Internal Scaffold Architecture Designs using Lindenmayer Systems,” Computer-Aided Design and Applications, vol. 4, no. 1–4, pp. 395–403, Jan. 2007. | spa |
dc.relation.references | M. Bashoor-Zadeh, G. Baroud, and M. Bohner, “Geometric analysis of porous bone substitutes using micro-computed tomography and fuzzy distance transform,” Acta Biomaterialia, vol. 6, no. 3, pp. 864–875, Mar. 2010. | spa |
dc.relation.references | L. Podshivalov, C. M. Gomes, A. Zocca, J. Guenster, P. Bar-Yoseph, and A. Fischer, “Design, Analysis and Additive Manufacturing of Porous Structures for Biocompatible Micro-Scale Scaffolds,” Procedia CIRP, vol. 5, pp. 247–252, Jan. 2013. | spa |
dc.relation.references | S. J. Hollister, R. A. Levy, T.-M. Chu, J. W. Halloran, and S. E. Feinberg, “An image-based approach for designing and manufacturing craniofacial scaffolds,” International Journal of Oral and Maxillofacial Surgery, vol. 29, no. 1, pp. 67–71, Feb. 2000. | spa |
dc.rights | Atribución-NoComercial-CompartirIgual 2.5 Colombia | * |
dc.rights.uri | http://creativecommons.org/licenses/by-nc-sa/2.5/co/ | * |
dc.subject.keyword | Bone scaffold | spa |
dc.subject.keyword | Reaction-diffusion models | spa |
dc.subject.keyword | Additive manufacturing | spa |
dc.subject.keyword | Porous structures | spa |
dc.title | Geometric and mechanical properties evaluation of scaffolds for bone tissue applications designing by a reaction-diffusion models and manufactured with a material jetting system | spa |
dc.type.category | Generación de Nuevo Conocimiento: Artículos publicados en revistas especializadas - Electrónicos | spa |
Archivos
Bloque original
1 - 1 de 1
Cargando...
- Nombre:
- Geometric and mechanical properties evaluation of scaffolds for bone tissue applications designing by a reaction-diffusion models and manufactured with a material jetting system.pdf
- Tamaño:
- 2.88 MB
- Formato:
- Adobe Portable Document Format
- Descripción:
- Artículo WOS
Bloque de licencias
1 - 1 de 1
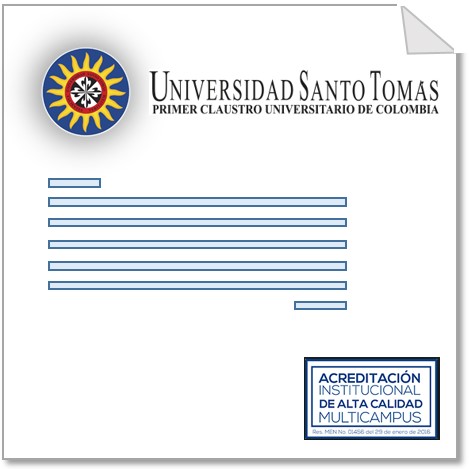
- Nombre:
- license.txt
- Tamaño:
- 807 B
- Formato:
- Item-specific license agreed upon to submission
- Descripción: